
Cassini Swarm



Using solar power is not practical for spacecraft farther from the Sun than the orbit of Jupiter. Since the mission is to the orbit of Saturn, solar panels cannot be used effectively. As an alternative power source, RTGs provide electricity and heat that can enable spacecraft to undertake scientific missions to environments beyond the capabilities of solar power, chemical batteries and fuel cells.
The Multi-Mission RTG (MMRTG) is the newest generation of RTGs and is recommended for this mission. When the Cassini mission was launched, RTGs were limited to vacuum environment applications only. Since there was no available RTG that could function within Titan's atmosphere, the Huygens probe was instead powered using a battery and Radioisotope Heater Units (RTUs), limiting its lifespan to 90 minutes on Titan's surface. A main advantage of an MMRTG is it works reliably for long periods of time in both vacuum and planetary atmospheres. By using an MMRTG in the CubeSats, data can be gathered for a minimum of six years [1].
The MMRTG should be the primary source of power for the orbiter and CubeSats but lithium-ion batteries will be used to power subsystems during launch.
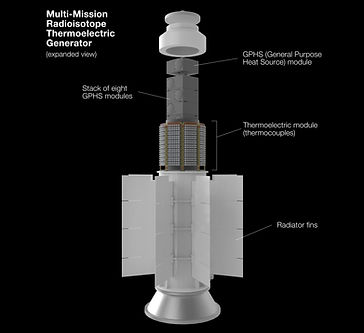
Who else has used RTGs?
Past operational and test experience of RTGs indicate good reliability in outer space. The recommended MMRTG has seen successful deployment in the Mars Science Laboratory Rover Curiosity.
Power Systems
Spacecraft operating in the outer solar system, where sunlight is too weak to produce sufficient power, rely on radioisotope thermal generators (RTGs) as a main energy source.

Figure 2. MMRTG MODEL
Risks involved with RTGs
Radioactive Contamination
RTGS pose a risk of radioactive contamination. If the container holding the fuel is destroyed, the radioactive material contained within will spill and may contaminate the environment. However, this event is not likely with current RTG cask designs. According to NASA’s Radiological Accident Impact Analysis on the risk associated with the Cassini mission, the overall chance of an accident occurring during the pre-launch, early launch, or late launch segments of the mission was 2.8x10^-2 [3]. In addition, the DOE conducts extensive safety testing to assure that the systems would be safe under all accident conditions, including accidents occurring on or near the launch pad and orbital reentry accidents.
Past Accidents with RTGs
On April 21, 1964, the Transit 5-BN-3 mission was aborted because of a launch vehicle failure resulting in burnup of the RTG during reentry. This resulted in dispersal of the plutonium fuel in the upper atmosphere. Subsequently, the RTG design was changed to provide for survival of the fuel modules during orbital reentry.
On May 18, 1968, the Nimbus B-1 launch was deliberately self-destructed remotely because it showed signs of an erratic flight trajectory. The heat sources were recovered intact in about 300 feet of water off the California coast with no release of plutonium. The fuel capsules were reworked and the fuel was used in a later mission.
In April 1970, the Apollo 13 mission to the moon was aborted following an oxygen tank explosion in the spacecraft service module. Upon return to Earth, the Apollo 13 lunar excursion module with a SNAP-27 RTG on board reentered the atmosphere and broke up above the south Pacific Ocean. The graphite reentry cask containing the heat source plunged into the ocean in the vicinity of the Tonga Trench, where the ocean depth is five to six miles. Atmospheric and oceanic monitoring showed no evidence of release of nuclear fuel.

MMRTG Specifications [3]

Figure 1. Curiosity's MMRTG
Why Use RTGs?
Further Motivating Advantages
(a) Independence of the distance to the sun and orientation with respect to the sun.
(b) Compactness (a 10 MW solar array would require solar panels that cover an area of 68 000 m^2 at the distance of Mars and 760 000 m^2 at Jupiter and their size would render them impracticable) [2].
(c) Better mass and size parameters when used on unmanned spacecraft, beginning with a power level of several tens of kilowatts.
(d) The capability of providing a power level two to three times greater with the NPS mass depending relatively weakly on the power improvement.
(e) Resistance to the earth’s radiation belts.
(f) The possibility of combining nuclear power with electrical thrusters to give the highest efficiency of specific impulse for thrust and of building power/propulsion systems on this basis to allow launch of payload masses two to three times greater than those possible with conventional chemical propellant orbital boosters. This can be achieved while supplying 50–100 kW of electrical power and more for onboard instrumentation over periods of 10 years or more [1].
References
[1] http://saturn.jpl.nasa.gov/spacecraft/safety/fseis4.pdf
[2] Stanculescu, A.. The role of nuclear power and nuclear propulsion in the peaceful exploration of space. Vienna: International Atomic Energy Agency, 2005. Print.
Online Publication: http://www-pub.iaea.org/MTCD/publications/PDF/Pub1197_web.pdf
[3] http://www.lpi.usra.edu/opag/march_08_meeting/presentations/dudzinski.pdf