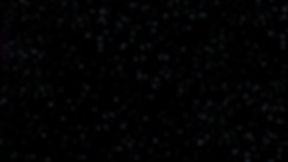
Cassini Swarm



CubeSats/Queen link
Data communication from CubeSats/Worker Drones to the Queen Bee occurs at 2040 MHz in S-band, while command signals from Queen Bee to CubeSats are sent at 2098 MHz. The modulation scheme used is Binary Phase Shift Keying (BPSK) with raised cosine pulse filtering with a rolloff factor of 0.4. BPSK is used because it requires less power. The data rate is 8192 bps. The Queen and cubesats usehalf-wave dipole antennas that will yield isotropic gain in the azimuthal direction since they are expected to operate in roughly the same plane as they are orbiting Titan.
The digital byte stream from each CubeSat is transmitted in a shared radio channel. This can result in collision of data streams from different transmitting worker bees. In order to avoid that Carrier sense multiple access with collision avoidance (CSMA/CA) is used.
CSMA/CA
CSMA/CA is a network multiple access method in which CubeSat senses the carrier before transmission and transmits only if the carrier is not in use. If the channel is busy, the CubeSat waits for the channel to be idle and backs off for a random time and subsequently transmits the data if the channel is idle.
To avoid the hidden node problem, a Request to send/Clear to send (RTS/CTS) mechanism is employed between each CubeSat and the Queen Bee.
Channel Coding
The atmoshperic attenuation in Titan is very small, less than 0.1 dB. So, relatively simple error correction code should suffice. The data stream between CubeSat and Queen is convolutionally coded using constraint length of 7 and rate 1/2 with generator matrix (171,133).
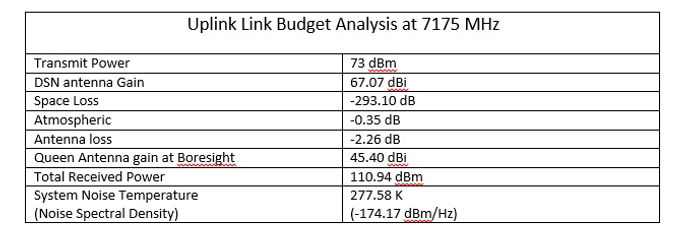
Communication with the Earth
The Deep Space Network (DSN) provides a communication link with the Queen Bee. DSN consists of three communication facilities separated 120 degrees apart in longitude around the Earth; at Goldstone, in California’s Mojave Desert; near Madrid, Spain; and near Canberra, Australia. The strategic placement of these facilities permits uninterrupted communication link between Earth and Queen Bee when the DSN tasks a transceiver for the mission.
The Queen bee can communicate with the DSN using either of the two stations described below using its 4-m High Gain Antenna (HGA).
34-m High Efficiency Station:
The DSN 34-m Antenna station contains three 34-m diameter high-efficiency (HEF) antennas. These antenna communicate in X-band or S-band. An orthomode junction for X-band is employed that permits simultaneous RCP or LCP operation. Under normal operation, transmission to and from Queen is done in opposite polarization.
WIth this station, the data rate is 1 gigabit/day.
70-m Station
Like 34-m HEF station, 70-m station can communicate in S-band and X- band. Queen communicates in X-band with a date rate of 4 gigabits/day with 12 hour track.
The details are provide in tables below.
Communication System
CubeSats and Queen orbit around the Titan in a similar fashion in "swarm". All the CubeSats transmit data to Queen which then gets processed and transmitted to Earth. Queen is tentatively at the center of the swarm and has line-of-sight communication link with all or most of the CubeSats.
Radio Frequency Systems
Surface mapping and precipitation measurement
The TitanTech SWARM system is designed to produce high-resolution images of both Titan’s surface as well as precipitation rates as a function of altitude. In order to achieve these twin goals, TitanTech queen and drones will operate the data collection radio frequency system at 3 distinct frequencies: a 6 GHz synthetic aperture radar system and two precipitation radar systems at 13.6 GHz and 35.5 GHz, each system building upon highly successful Earth based missions using similar technology.
Data Capture
Synthetic aperture radar (SAR) is a radar imaging method whereby an acquisition system uses its radar system and special backend processing to “stitch” together multiple radar images of a single target as seen from different vantage points. The key is to have the acquisition system moving and producing an equivalent “larger” aperture through time. While this allows a stand-alone radar system to develop high-quality images of a surface or target, it does not produce enough information to resolve 3D images.
Interferometric SAR (INSAR) is an extended technique of SAR where multiple acquisition systems that have been spaced apart are used to develop independent SAR images of a target. By taking images from different points, similar but different phase patterns of the same target are measured. The phase differences between SAR images resolve range ambiguities between the two images, in this case allowing for the calculation of height of surface features on Titan.
Titan is the only other nearby stellar body with an Earth-like water cycle, substituting methane for H20. Mapping the surface will provide insight on the role of weather patterns on distant planets and moons and can provide confirmation or new information in regards to the role proximity to the sun has on climate. Furthermore, it is believed that the atmosphere on Titan is similar to that of a prehistoric Earth before photosynthetic organisms began to populate and produce oxygen to help create the atmosphere that we have today. Mapping the surface could allow us to take a glimpse into Earth’s past and determine what kind of geological formations were possible at that time.
As has been mentioned, Titan is distinctly characterized by precipitation, specifically a methane cycle that closely mimics Earth’s water cycle. Utilizing similar technology as used in the Global Precipitation Measurement mission, a dual band radar will be deployed to offer both high and low resolution of precipitation at either 250m or 500m altitude resolution. Furthermore the system is predicted to be able to provide precipitation rates at a resolution of 0.2 mm/hr or better. This precise information will allow scientists on Earth determine the effects that the lower gravity and differing atmosphere has on rainfall in general. It has also been hypothesized that due to slower rainfall rates that much of the methane never reaches parts of the surface, but instead evaporates mid-fall to fall again. High resolution data from the clouds to the surface of Titan could help answer this question and will be critical to planning for any rover type missions that might occur on Titan in the future.
Technical Specifications
The satellite cloud will orbit at approximately 700km. All data acquisition system will operate in a circular-multistatic approach with the “Queen” operating as the transmitter and backend processor for the data. This will keep the power consumption on the cube-sats minimal. The basic flow will look as follows:
-
The Queen transmits a pulse down towards the surface.
-
Depending on the frequency and ambient condition on Titan the energy is reflected back by either rain or the surface
-
The cube-sats receive the different radar signatures
-
The cube-sats transfer the raw data to the Queen to be processed
-
The Queen processes the INSAR images and rainfall data and then stores it to disk until a reliable uplink can be established with Earth
Each of the radar systems is built on already existing work developed for Earth-centric systems: the Shuttle Radar Topography Mission (SRTM) and the Global Precipitation Measurement Mission (GPMM). The SRTM was utilized on an 11-day mission in February of 2000. A mast with an antenna was extended from Space Shuttle Endeavour and was used to capture SAR images of earth while a similar antenna was based on the shuttle creating what was at the time the most complete global topographic map ever made. While the SRTM used two frequencies, a C- and an X-band radar, the TitanTech mission will use only a C-band radar due to space and energy constraints on the satellites.
For the Titan topographic mission, the Queen will have a fixed beam array with a peak gain of approximately 42.4 dBi of gain. This will allow the array to cut a swath on the surface of Titan that is 5km by 100km. The cube-sats will have approximate gains of 5-10 dBi (HPBW of ~60 deg) in order to receive signals regardless of distance from the Queen. Friis transmission equation predicts that the worst power level to be expected for a given transmit power from Queen to cubesat is:
It is to be noted that this is assuming a perfect RCS for the Titan surface.
For the Titan rainfall mission, the Queen will have a similar fixed beam array with peak gains of 41.6 dBi and 38.5 dBi for Ka- and Ku-bands respectively, resulting in swaths of 5 km by 120 km and 5 km by 250 km. Resulting power received normalized to power transmitted levels are as follows:
Note, this is a worst case if there is no rain and the waves travel all the way to the surface and back. Furthermore, a loss factor has not been introduced as Titan’s atmosphere is primarily molecular methane and nitrogen, neither of which introduce any significant losses at the frequencies to be used.
Potential Pitfalls
Potential issues that might arise and that need to be accounted for in utilizing systems like these are:
-
Phase locked loops must be employed between the Queen and the cube-sats to utilize the radar data
-
Relative positions of cube-sats with respect to the Queen must be known within, at maximum, a sixteenth of a wavelength (λ/16) to make any appreciable calculations based off of the data.
-
Doppler shift must be within the bandwidth of the system or design receiver systems on cube-sats to be at the Doppler shifted value given the orbital height of the cloud
All of these issues can be addressed in either preliminary design stages or in the range finding system utilized by the Queen to locate all the cube-sats.
References:
[1] http://www2.jpl.nasa.gov/srtm/index.html
[2] http://science.nasa.gov/missions/trmm/
[3] http://www.space.com/11154-saturn-titan-methane-rain-desert.html
[5] http://pmm.nasa.gov/node/243
[6] Lorenz, R. D. "Raindrops on Titan." Advances in Space Research 15.3 (1995): 317-320.




